Chemistry is the science of change. But why do chemical reactions take place? Why do chemicals react with each other?
The answer is in thermodynamics and kinetics. These two are arguably the most important concepts to grasp in chemistry.
But sometimes, even after several years of basic chemistry education, it is not easy to form a clear picture on how they govern reactivity!
Tutorial Review: Contents and Introduction
In this tutorial, we will try to introduce and summarize what these two concepts are, and their implications in chemical reactivity. This is obviously an introduction, intended for chemistry students of all levels.
For sure, there are more comprehensive explanations out there, and if you want one, go grab any of the best-known general chemistry books, or a more specific organic chemistry textbook. However, we have found that there are not many short explanations out there available for the general scientific public. This is somehow worrying, since, without a basic understanding of thermodynamics and kinetics, there is no way to understand the basic principles of reactivity. And without understanding reactivity, you are missing out on the most important part of chemistry.
But if you really want to dive on physical chemistry concepts as such, unfortunately you will find that most books can be impenetrable without a basic understanding of maths, physics and chemistry itself.
This is what we want to fix with this short tutorial. To give you a general overview on why chemical compounds react. We want to break the gate-keeping that has always been going on with physical organic chemistry!
As mentioned, this is intended to be brief. We will start off with the basic definitions, and hopefully make you go all the way through to understanding free-energy profiles of catalytic reactions.
Interested yet? Keep reading!
- A quick disclaimer: Since my background is in organic chemistry, I will base the explanations on simple organic chemical reactions, but most of the general principles apply to any kind of chemical reactivity.
- For most energy diagrams, the energy values are orientative, made up in order to explain the concepts.
Thermodynamics: The Energetic Stability of Molecules
Thermodynamics is the branch of physics that deals with heat, work and temperature, and their relation to energy, radiation, and physical properties of matter.
That is the definition of thermodynamics. As you can see, it is an incredibly broad concept. Let’s forget about it for now. How does thermodynamics dictate why do chemicals react?
Well, imagine every different molecule having an associated value for energy.
Some molecules will have larger energies and some others lower energies. Then consider that every chemical system has the tendency to go towards the point of lowest energy possible, in effect, the most stable point. This means that a molecule with high energy (less stable) will have a tendency (or “will want to react”) to transform into another molecule with lower energy (more stable).
Why is that? Because the process of going from a high energy state to a lower energy state releases energy, or heat, in what is called an exothermic process. This is what we call a thermodynamically-favored process, and it is basically what the laws of thermodynamics are telling us.
Thermodynamic Stability in Energy Diagrams
This is easier than it might sound. The image below illustrates what you just read. Molecule A can react in two ways: Absorbing heat it can be transformed into 1. Alternatively, it can evolve into 2 by releasing energy, in a thermodynamically-favorable manner. Of course, if these were the only two possible scenarios, all molecules of A would react to give 2, and stay there forever. But the picture is usually not that simple, and we will come back to this later.
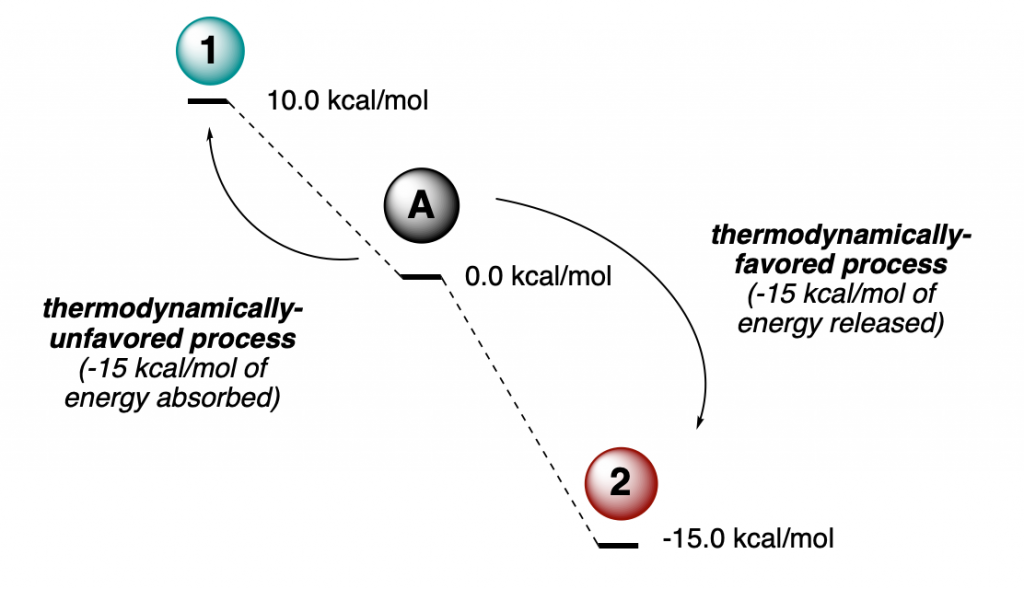
You might be looking at the picture above and still not get what we mean by higher or lower energy. Let me redraw it in a way you will probably have seen elsewhere, or been taught in school/college:
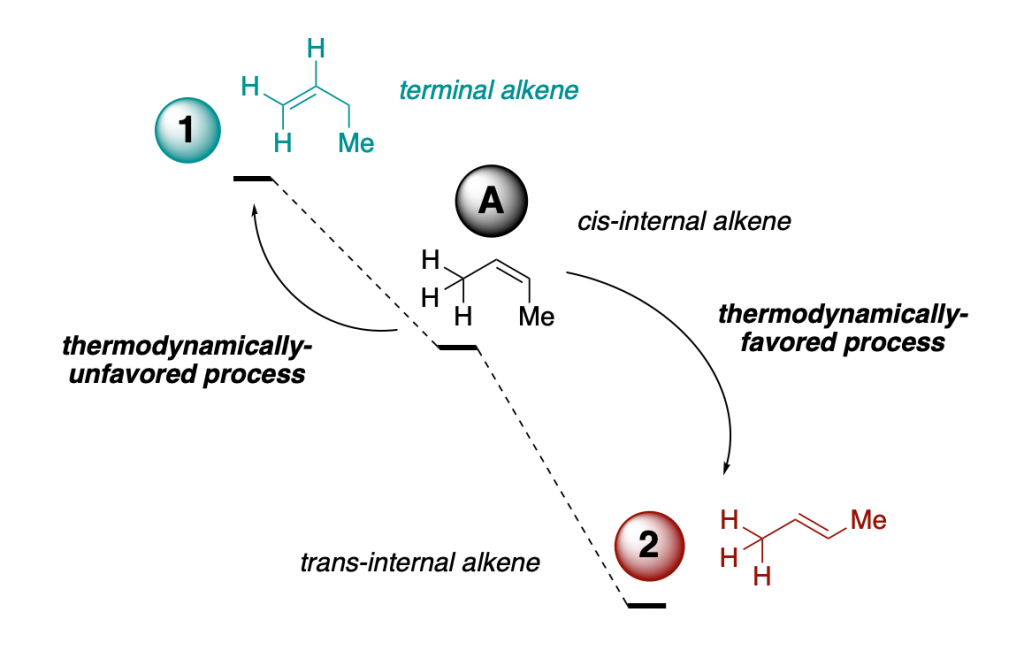
The scheme above resembles what you are always taught in introductory organic chemistry courses: more substituted isomers of alkenes are more stable (A and 2 vs. 1) and trans isomers are more stable than cis isomers (2 vs A).
What does this means? It means that, given the appropriate circumstances or conditions, both 1 and A would like to react to give 2. But we now that less stable alkenes such as 1 are perfectly inert, and can be handled and stored without worries. So, what is the deal here?
The answer is kinetics and activation barriers.
Kinetics: The Barriers of Chemical Reactivity
Before diving into kinetics, let me present another quick example of a thermodynamically-favorable process. An energy diagram of an Sn2 substitution reaction in this case.
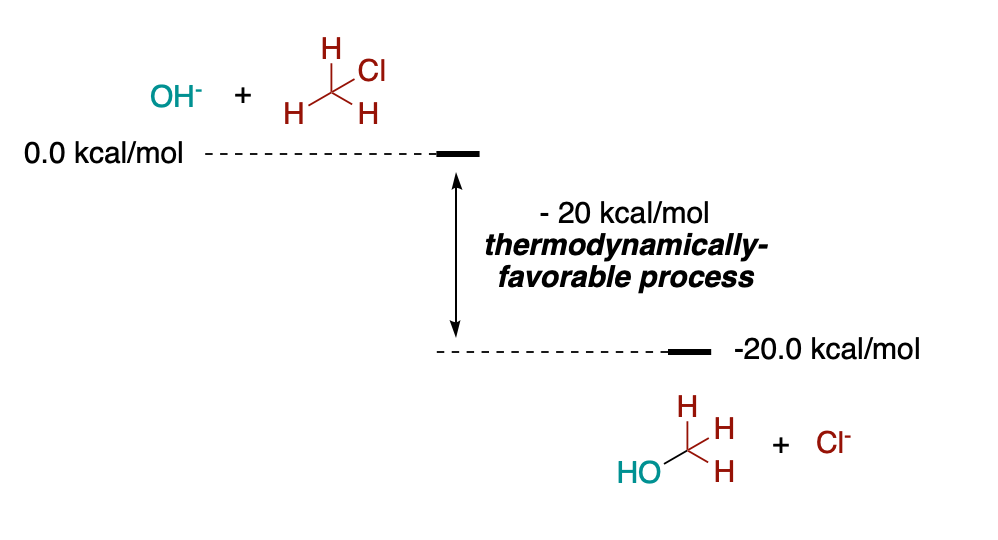
As you can see, if we set the zero in energy for a hydroxyde anion plus a chloromethane molecule (in energy diagrams you always set an energy for the whole system, not individual molecules), the total energy of the products of the corresponding Sn2 reaction (tert-butyl alcohol and a chloride anion) will be lower (about 20 kcal/mol lower!).
What does this mean? That the reaction is thermodynamically favorable, and in principle it will not take place the other way around.
But does this mean that, mixing hydroxide with chloromethane at any temperature will lead to the immediate formation of tert-butyl alcohol and chloride? Of course not! The rate at which the reaction proceeds will depend directly on the temperature, and if the temperature is low enough, the reaction will not take place at all, even though the process is thermodynamically favorable.
Why is that? Kinetics is the answer.
What Are Chemical Reaction Kinetics?
Chemical or reaction kinetics is the branch of physical chemistry that studies the rates (or speeds) of chemical reactions.
In summary, thermodynamics determines in what direction a chemical reaction proceeds, and kinetics determines the speed or rate at which that process occurs.
Of course, in the last scheme of the previous section, there was something missing. In a chemical reaction, reactant A does not simply transform into product B. Reactions take place through what we call transition states.
Transition states are intermediate structures between reactants and products of a chemical reaction step. They are usually higher in energy (less stable) than both the reactants and the products, and the energy difference between the reactants and the transition states, also known as activation energy, is the barrier necessary to overcome for a thermodynamically-favorable reaction to take place.
Why Do Chemicals React? Thermodynamics and Kinetics Combined
See below a now complete version of the free-energy diagram of the Sn2 substitution reaction. As you can see, the process is thermodynamically favorable, but a barrier or activation energy of 23.0 kcal/mol has to be overcome in order to reach the products.
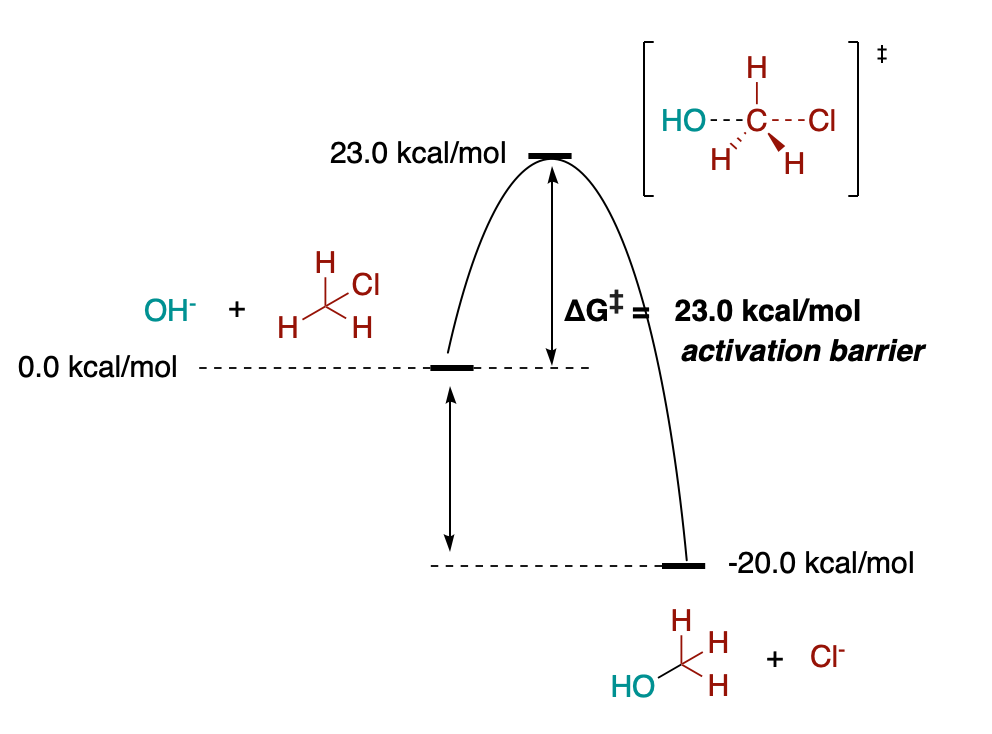
The larger the activation energy, the lower the speed or rate of a reaction at any given temperature. Usually, we set a limit of barriers of around 25 kcal/mol for reactions to proceed at a significant rate (this is, in several hours or days) at 25 ºC. Easy enough to remember.
Stability vs Inertness
No matter how thermodynamically favorable a process is, if the barriers to reach the corresponding transition are too high (say, higher than 30-40 kcal/mol), that chemical reaction is not going to take place under regular conditions.
This allows us to make a clarification between stability and inertness as properties of chemicals.
Stability is a thermodynamic concept, while inertness is a kinetic concept.
- A compound is stable, if it is relatively low in energy (compared to the molecules to which it may interconvert into). The opposite would be unstable, high in energy.
- On the other hand, we say that a compound is kinetically inert if in order to react it has to overcome large activation barriers.
A compound can be both unstable and inert. That is why we can handle and store thermodynamically unstable primary alkenes such as 1-propene without them isomerizing to more-stable secondary alkenes such as cis- or trans-2-propene (see the first two schemes).
But we can trick kinetics! Catalysts can be used to lower the activation energy of chemical transformations, allowing them to proceed more rapidly, or simply to proceed at all!
- Note: Theoretically, no matter how high the activation energy of a process might be, we say that it is always taking place at a certain rate. However, if the energy barriers are higher than, say, 50 kcal/mol, the rate or speed of the reaction would be so low that it would take many many years before we can detect a significant conversion.
Catalysis: Lowering the Barrier!
Now that we know why chemicals react, let me explain how we chemists try to override the system and make activation barriers lower.
A catalyst is a chemical entity (a molecule, a salt, a coordination complex…) which speeds up a chemical reaction. It also can unlock new reactivity pathways and make reactions work that would not be possible otherwise.
Let’s take a specific classical example. The electrophilic aromatic substitution of benzene with molecular bromine (Br–Br). This reaction is traditionally carried out using a Lewis acid as catalyst, such as iron tribromide.
But let us imagine first a catalyst-free version of the process, which I am certain can occur if you mix together benzene and bromine, and heat it up enough.
How Thermal, Catalyst-Free Reactions Occur
The first step of this reaction is the formation of the well-known Wheland intermediate. An intermediate (not to be confused with a transition state, which rather connects intermediates together) is a reactive chemical species which is formed in one of the steps in the middle of a chemical reaction of A leading to B, as intermediate point. For benzene to be transformed into bromobenzene, it has to pass through this intermediate species. Intermediates can rarely be isolated, since they usually are both thermodynamically unstable and kinetically reactive.
In any case, the reactants have to overcome a high activation barrier of 30 kcal/mol. Once the temperature is enough for this to take place, the rest of the process has lower activation barriers, and takes place downhill to give reaction products in an overall thermodynamically favorable process (exothermic by -11 kcal/mol).
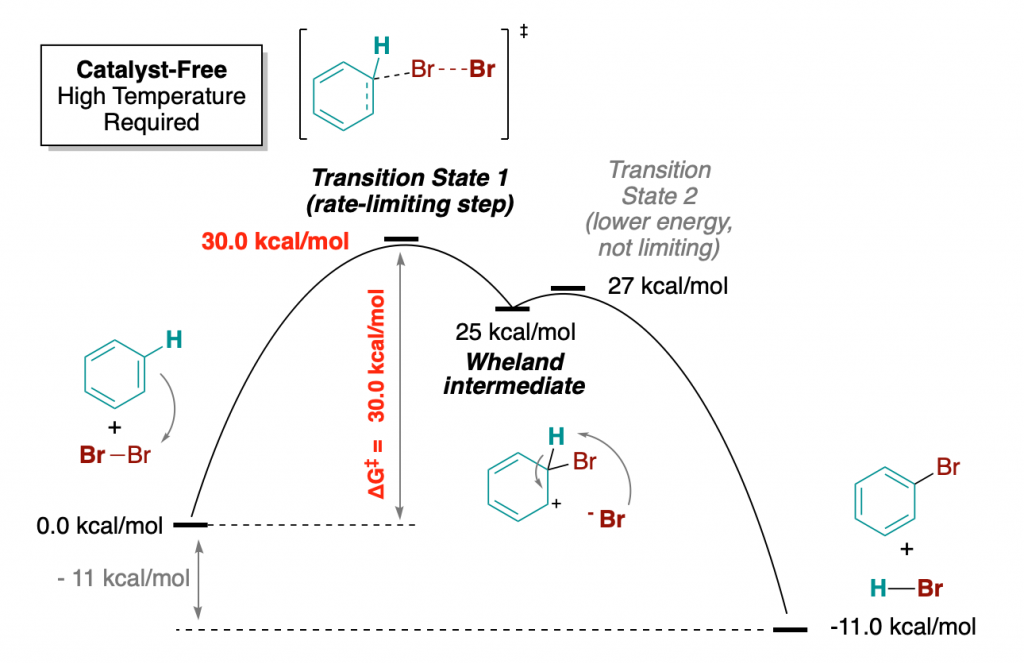
But we can speed things up with a catalyst!
Lewis Acid-Catalyzed Electrophilic Aromatic Substitutions
By adding a catalyst to the mixture, we can access new transitions states, which are more stable, and hence lower in energy. And what happens when the transition state of the rate-limiting step of a reaction is lower in energy? That the activation barrier of the whole process is much lower!
This is basically the role of FeBr3 (the catalyst) of this reaction: stabilizes transition states and intermediates. Now the activation barrier to reach the first transition state is much lower (20 vs 30 kcal/mol), allowing the reaction to take place under mild conditions.
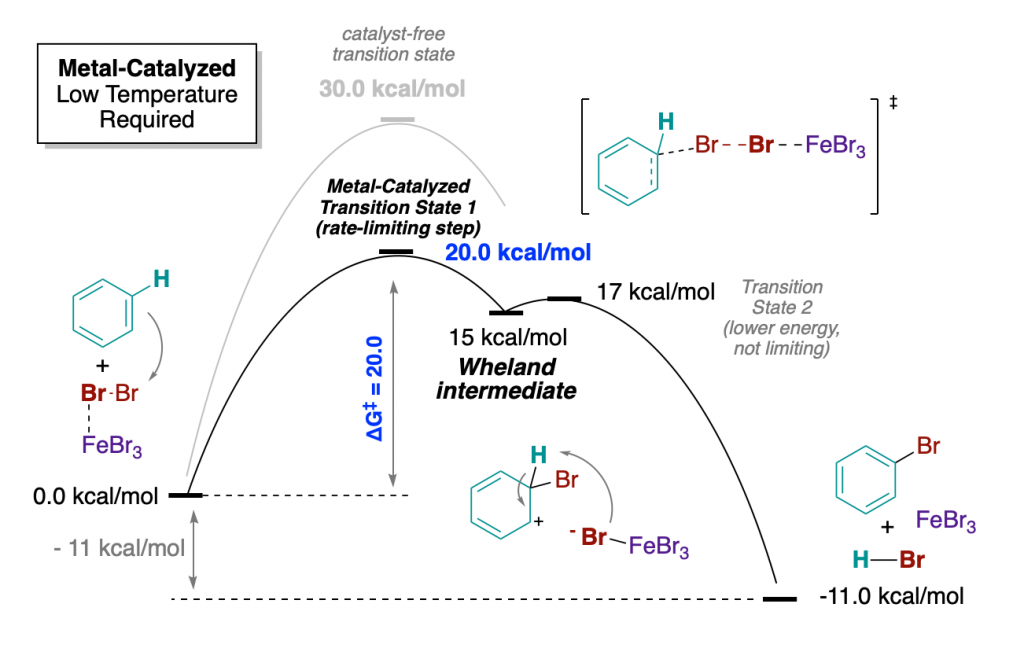
Also, note that FeBr3 is recovered unreacted with the products. This is another feature of catalysts: they can be recovered and re-enter another reaction cycle. This is why they are often employed in sub-stoichiometric amounts (this is, less than one mole of catalyst is enough to drive full conversion of one mole of starting material).
But as we have already mentioned, thanks to catalysis we not only can lower activation barriers that would need totally unpractical temperatures. We can also unlock reactions that would take hundreds of years to complete by themselves. We can also achieve completely new selectivities, and develop new chemical processes.
I work in catalysis myself, and I can tell you this is one of the most important, exciting and active fields of chemistry.
This is the end of this tutorial review, and I hope it has helped you to get a clearer picture of why chemical reactions take place and what leads chemicals to react. We certainly covered concepts you have to master if you are learning chemistry at any level!
Post a Comment